Experimental details
We want to investigate the quantum phenomena and correlations in a degenerate Fermi gas of 6Li. For this we have to cool down the atoms to a few tens of nanokelvin. For this purpose, different techniques from the field of laser cooling are combined.
The basic prerequisite for this is that the atoms to be cooled are present as a gas. For this reason, crystalline lithium is converted into the gaseous state of aggregation at approx. 400 °C in an oven. The lithium gas then leaves the oven through its aperture forming a beam of lithium atoms.
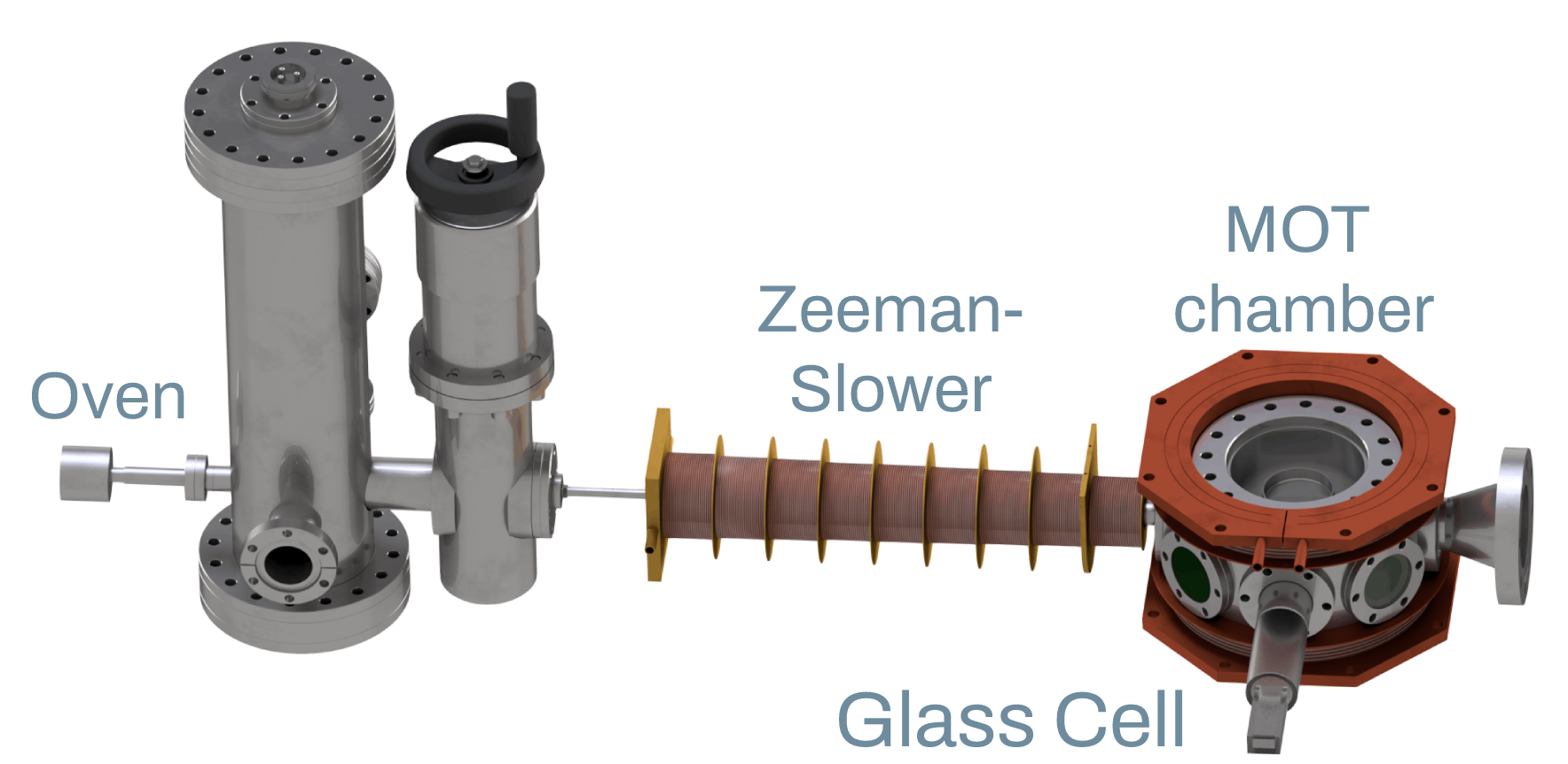
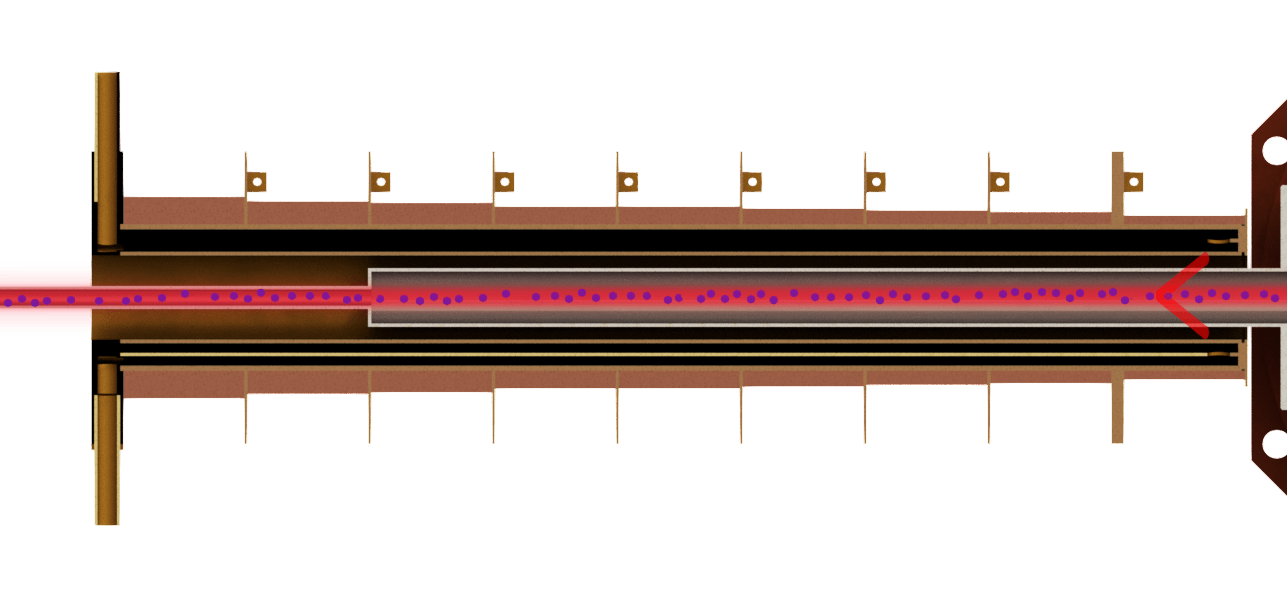
In the first step of the cooling process, these lithium atoms are decelerated in a Zeeman slower. Our Zeeman slower consists of a red detuned laser beam of fixed frequency, which is propagating opposite to the direction of the atoms motion, and a locally varying magnetic field generated by nine different coils. By absorbing photons from the laser beam and re-emitting them spontaneously each atom receives many momentum kicks from the photons which eventually slows it down. The external magnetic field created by the nine coils helps us to keep the laser resonant to the atoms. It induces a Zeeman shift of the atomic line. This shift perfectly compensates for the changing Doppler shift that the atoms experience when being slowed down. This way we can make the laser frequency always resonant to the atomic transition which leads to the optimal deceleration of the atoms.
At the position where the final velocity of the atom beam is almost zero, we trap the atoms with a magneto-optical trap (MOT). The MOT consists of a spatially varying magnetic field and two opposing laser beams for each spatial direction. This leads to a position and velocity dependent scattering rate for the atoms. The system behaves similar to a damped harmonic oscillator. If an atom moves away from the center of the MOT, it experiences a momentum kick in the direction of the trap center by the scattering with the light. The MOT also dampens the velocity of the atoms which leads to a further cooling. This way the trapped atoms in the MOT are cooled to a temperature of several hundred microkelvin. This is close to the Doppler cooling limit of ~150 µK for the D2 line of 6Li.
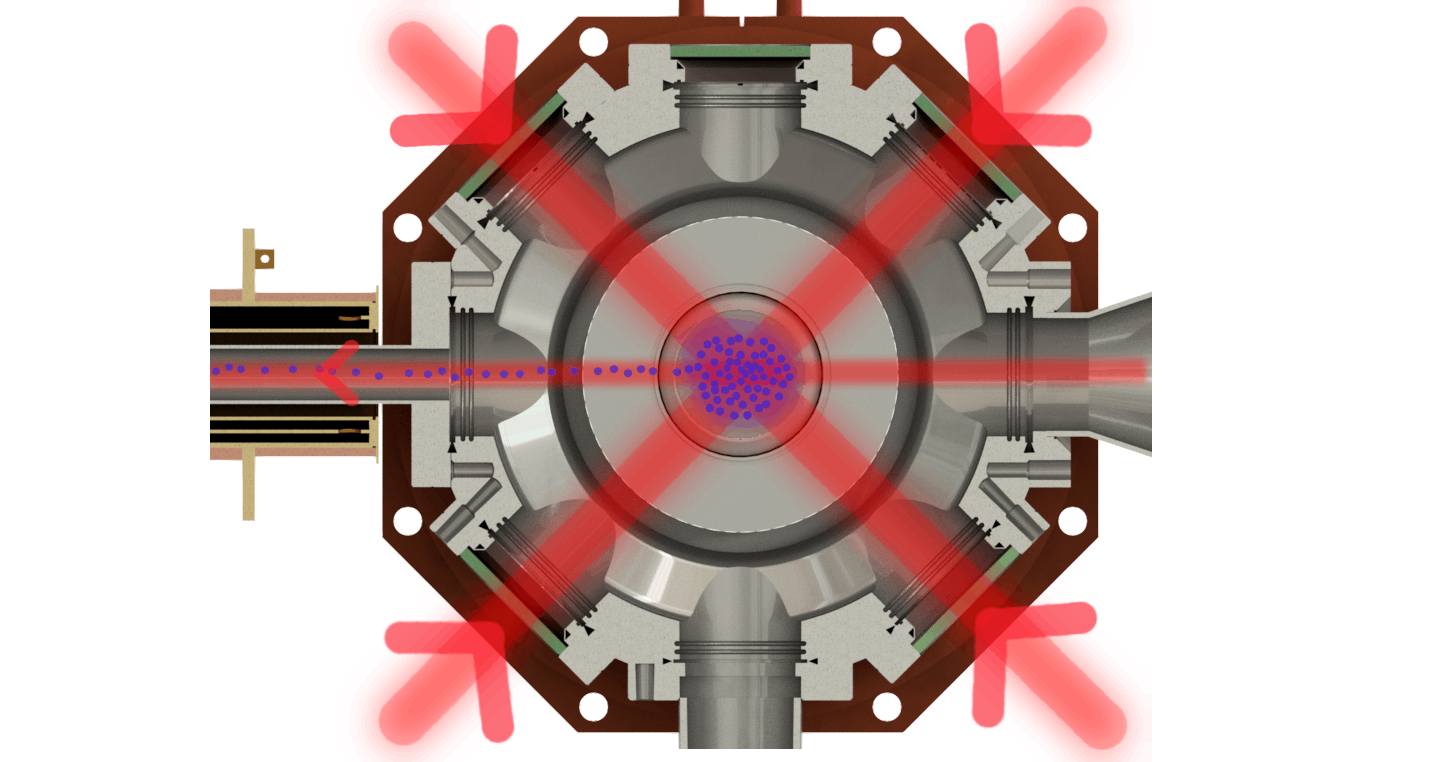
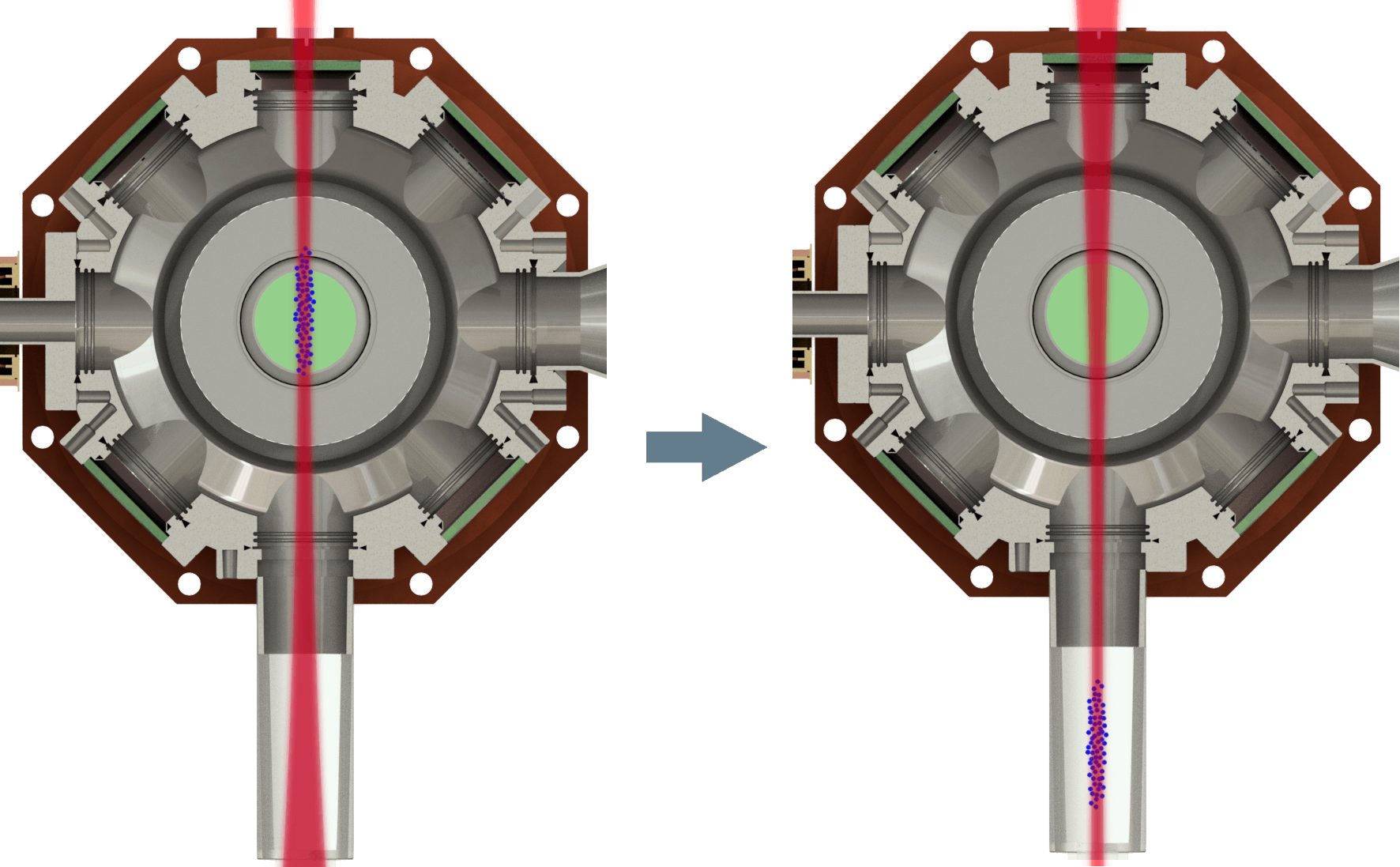
In order to cool the atoms even more, they are loaded into an optical dipole trap (ODT). A laser which is strongly detuned from the atomic transition induces an electric dipole moment in the electron shell of the atoms. The induced dipole moment interacts with the electric field of the laser creating a force. The direction of the resulting force depends on the sign of the detuning. In our case the light from the dipole trap laser is red detuned to the atomic transition. Therefore the atoms experience an attractive force towards the focal point of the laser beam. After loading the atoms from the MOT into the dipole trap, the focus of the dipole trap laser is shifted to the glass cell.
When the atoms arrive at the final position in the glass cell evaporative cooling is started. The intensity of the dipole laser is slowly ramped down. This way the hottest atoms are able to leave the trap potential and we remain with a colder gas. In contrast to the MOT steel chamber, the glass cell allows much better optical access to the atoms. Here most of our experiments are carried out.
After the evaporative cooling the atoms have a temperature of only a few tens of nanokelvin and the atomic cloud is ready for the desired experiment (see list of papers on the previous page). With laser beams and magnetic field coils, the atoms in the glass cell can be prepared depending on the requested experiment. To prevent the atoms from colliding with the background gas and being heated up during the cooling process, the whole experiment is performed in an ultra-high vacuum (10-10 mbar).
The detection of the atoms is done by absorption imaging: Using a resonant laser which is absorbed by the atoms, a shadow image of the atomic distribution is taken with the help of a CCD camera. This allows us to reconstruct the exact density distribution of the atoms.