Gas Phase Model Systems for Catalysis
Small metal, metal-oxide, and metal-sulfur particles often represent the catalytically active centers (i.e., the location at which the actual chemical transformations take place) of heterogeneous and homogeneous catalysts as well as biocatalysts. In oder to desgin new tailor-made effective, selective, and energy efficient catalytic materials for specific reactions it is mandatory to understand the elementary processes of the catalytic reactions on a strictly molecular level. Towards this goal we employ small free clusters as model systems to mimic the catalytically active centers. Whith this approach we aim to gain fundemental insight into the kinetics and energetics of catalytic reactions as well as to elucidate bond activation/formation and charge transfer processes on a molecular level. In particular we focus on the influence of cluster specific properties (cluster size, charge state, composition, ligands) on the reactivity, catalytic activity, and selectivity. Furthermore, we are interested in coadsorption phenomena which often represent the first and rate-determining reaction steps in catalytic reactions and might even lead to catalyst poisoning.
More details can be found in:
- Phys. Chem. Chem. Phys. 14, 9255 (2012)
- Bunsenmagazin 15, 283 (2013)
- Chemical reactivity and catalytic properties of size-selected gas phase metal clusters, in ‘The chemical physics of solid surfaces (Vol. 12): Atomic clusters: From gas phase to deposited’, edited by D. P. Woodruff, Elsevier (2007).
- Gas Phase Model Systems for Catalysis - GPMC 2018
Research Projects
Old materials with new functionality - Interstellar MgSiO clusters as novel and cheap catalysts
Magnesium containing silicate dust is an important component of the interstellar medium which not only plays a significant role in the life cycle of cosmic matter but also as a catalyst for the formation of various molecules. Most interestingly, there are indications that up to 10% of the mass fraction of silica grains arise from a large population of very small particles with diameters of less than 1.5 nm. However, the mechanism leading to the formation of such particles is still largely elusive, although water molecules have been suggested to play a fundamental role in this process. If we can experimentally proof this growth process and elucidate its mechanistic details, we will also be able to use this knowledge to address a fundamental problem of the modern energy storage economy on earth: the development of novel catalysts for sustainable hydrogen production.
The sustainable hydrogen production (i.e. independent of fossil fuels) represents the basis for the future large scale application of hydrogen fuel cells. The theoretically predicted properties of the small MgSiO clusters to deprotonate water and to catalyze the subsequent hydrogen evolution reaction render them promising materials for such catalyst. Furthermore, such MgSiO cluster based catalyst would be very cheap materials since the elements Mg, Si, and O are not only highly abundant in the interstellar space but also on earth. However, the potential of MgSiO clusters for such applications has not been investigated so far and only little is known about the structure and their chemical properties. Thus, the goal of the current project is to gain a fundamental insight into the astrochemically and astrophysically highly relevant cluster growth process of MgSiO clusters via water adsorption and to investigate the potential of MgSiO clusters for applications as novel and cheap catalysts for hydrogen evolution at the same time.
CO2 hydrogenation by copper oxide clusters
In the framework of the European Training Network CATCHY we will investigate the properties of copper oxide and doped copper oxide clusters for CO2 hydrogenation. More information on this project will follow soon.
Modeling the active center of photosystem II by small (calcium-)manganese oxide clusters
The direct conversion of solar energy into storable fuels represents one of the main challenges in catalysis research nowadays. In this respect, the oxidation of water to molecular oxygen and molecular hydrogen is of particular interest since the produced H2 can be directly utilized in hydrogen fuel cells. In biological systems water oxidation is realized in photosystem II (PS II). Recent X-ray diffraction investigations revealed that the detailed structure of the active water oxidizing center (WOC) of the PS II consists of a near cubic CaMn3O4 unit tethered to a fourth manganese atom through additional oxo bridges.
In this project we aim to mimic this catalytically active center of photosystem II aiming at uncovering phenomena which could aid the design of artificial water-splitting molecular catalysts. In a first step we have employed free manganese-oxide clusters as molecular models. The interaction of these ligand-free inorganic manganese oxide nano-clusters with water was investigated in an ion trap and, in conjunction with first-principles calculations, new mechanistic insight into the water splitting process could be obtained. Furthermore, we have performed vibrational spectroscopy on these clusters which provided direct insight into the ability of these clusters to dissociate several water molecules.
Currently we are increasing the complexity of the investigated clusters step by step via (1) increase of the cluster size, (2) addition of heteroatoms, and (3) addition of suitable ligands to mimic the cluster environment in PS II. With this hierarchical approach we aim to (1) provide increasingly complex model systems and (2) tune the reactive and catalytic properties of the biomimetic systems.
Project related publications:
- J. Phys. Chem. A 124, 4990 (2020)
- J. Phys. Chem. A 124, 1561 (2020)
- Phys. Chem. Chem. Phys. 21, 23922 (2019)
- Angew. Chem. Int. Ed. 131, 5892 (2019)
- Int. J. Mass Spectrom. 433, 7 (2018)
- Phys. Chem. Chem. Phys. 18, 15727 (2016)
- Angew. Chem. Int. Ed. 127, 15328 (2015)
- J. Phys. Chem. C 119, 10881 (2015)
Modeling the active active centers of iron-sulfur proteins
Iron-sulfur proteins represent an important class of proteins which are ubiquitous in all living organisms. First discovered in the 1960s the number of known iron-sulfur proteins has steadily been growing. These non-heme iron-containing proteins all comprise cofactors with iron atoms that are ligated to inorganic sulfur or cysteine sulfur and thus form iron-sulfur clusters. Besides their role as biological sensors, structural stabilizers, sulfur donor, as well as regulator of gene expression and enzyme activity, the main function of iron-sulfur clusters is the electron transfer in biochemical redox reactions. For this purpose nature has developed iron-sulfur clusters of different size and composition.
As manifold the functionality and the active sites of iron-sulfur enzymes are as manifold are the open questions, unsolved problems, and obstacles which have to be removed for the application of iron-sulfur clusters as technical catalysts. Towards this goal, we have started a new research program which aims to prepare and study bare and ligated iron-sulfur clusters in the gas phase to gain a fundamental insight into the intrinsic reactive and catalytic properties of these clusters.
So far our work focused on the properties of non-ligated iron-sulfur clusters for nitrogen fixation, the activation of water, and the activation of hydrocarbons. A further important aspect that has recently been studied is the oxygen sensitivity of the clusters. In all these studies we focused on changes in the reactive properties with cluster size.
Project related publications:
Activation and conversion of hydrocarbons by gold clusters
The selective activation of the strong C-H bond of methane and other hydrocarbons and the subsequent catalytic conversion into more valuable products represents one of hte major challenges in catalysis research. A molecular level insight into the mechanism of methane activation is therefore highly desirable and can contribute decisively to the successful formulation and implementation of versatile and selective catalytic reaction pathways as well as the future targeted design of novel catalytic materials. During the last years we have focused our research activities on the investigation of small gold, palladium, and binary gold-palladium clusters and their reactive and catalytic properties for the activation and conversion of hydrocarbons.
Selected project related publications:
- J. Am. Soc. Mass Spectrom. 30, 1895 (2019)
- Int. J. Mass Spectrom. 435, 241 (2019)
- J. Phys.: Cond. Mat. 30, 504001 (2018)
- Angew. Chem. Int. Ed. 56, 13406 (2017)
- ACS Catalysis 5, 2275 (2015)
- Angew. Chem. Int. Ed. 49, 980 (2010)
Selective CO methanation by ruthenium clusters
With respect to applications in transportation and decentralized small scale applicances the low temperature proton exchange membrane fuel cell (PEMFC) has gained increasing importance. The hydrogen feed gas for PEMFCs is often generated by reformation of fossil fuels which, however, results in the formation of PEMFC poisoning CO as an undesired byproduct. Thus, the development of cost-efficient techniques to improve the feed gas quality (minimization of the CO fraction) represents an important research field. One promising procedure for CO removal is the catalytic methanation (reaction of CO with H2 to form CH4 and water). However, feed gases usually also contain about 10% of CO2 which can be catalytically converted to CH4 or CO, respectively, which must be avoided. Thus, the selective CO methanation is essential for the future viability of the PEMFCs. For this purpose oxide supported ruthenium particle catalysts have been shown to be very promising materials.
We have employed free ruthenium clusters as model systems to discover the origin of the outstanding performance of supported sub-nanometer ruthenium particles. Using ion trap mass spectrometry in conjunction with first-principles density functional theory calculations three fundamental properties of these clusters are identified which determine the selectivity and catalytic activity: (i) high reactivity toward CO in contrast to inertness in the reaction with CO2; (ii) promotion of cooperatively enhanced H2 coadsorption and dissociation on pre-formed ruthenium carbonyl clusters, i.e. no CO poisoning occurs; and (iii) the presence of low coordinated Ru-atom sites, which are particularly active for H2 coadsorption and activation. Only recently, we have shown that the properties of the ruthenium clustes are maintained inside the pore of an ZSM-5 zeolite.
Project related publications:
ChemCatChem (2020) doi: 10.1002/cctc.202000716
Angew. Chem. Int. Ed. 53, 5467 (2014)
J. Phys. Chem. A 1118, 8356 (2014)
Low temperature CO oxidation by palladium clusters
The oxidation of carbon monoxide to carbon dioxide (for example in the automotive catalytic converter) is not only of scientific but also of environmental importance. However, the main problem of today`s three way catalytic converters is the rather high operation temperature of at least 570 K inhibiting the conversion of pollutants in the start-up period. Thus, it is of utmost importance to develop new low temperature CO oxidation catalysts.
We recently investigated the catalytic activity in the CO combustion reaction of small palladium cluster in the size range of two to seven atoms. Similar to extended palladium single crystals and supported nanoparticles the catalytic activity was found also in the case of the free palladium clusters to be largely determined by the fast adsorption and dissociation of molecular oxygen and the binding strength of carbon monoxide. In particular Pd4+, Pd5+, and Pd6+ could be identified to actively catalyze the CO oxidation, with Pd6+ being most active. The outstanding activity of this cluster was found to arise from a considerably reduced CO bond strength on Pd6+ in conjunction with the ability to dissociatively chemisorb O2 very fast. This prevents CO poisoning at room temperature and below and enables effective low temperature CO oxidation. With these studies we were able to show that free clusters cannot only serve as model systems for mechanistic studies of catalytic reactions, but may also provide new possibilities for the rational design of effective low temperature CO oxidation catalysts by changing the number of constituent atoms.
Project related publications:
J. Am. Chem. Soc. 134, 20654 (2012)
Phys. Chem. Chem. Phys. 14, 9364 (2012)
ACS Catalysis 5, 2275 (2015)
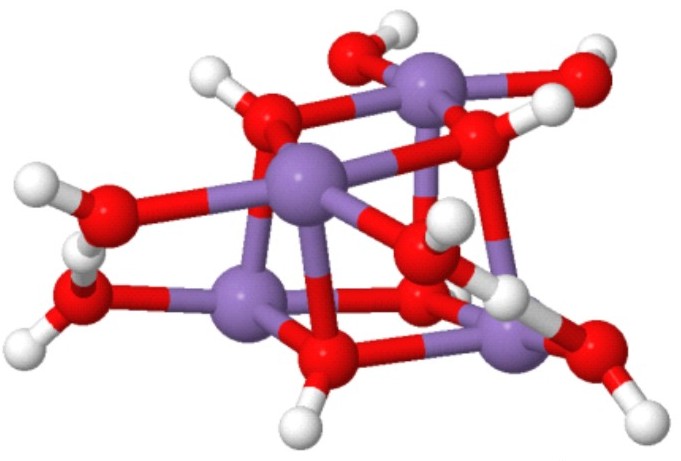
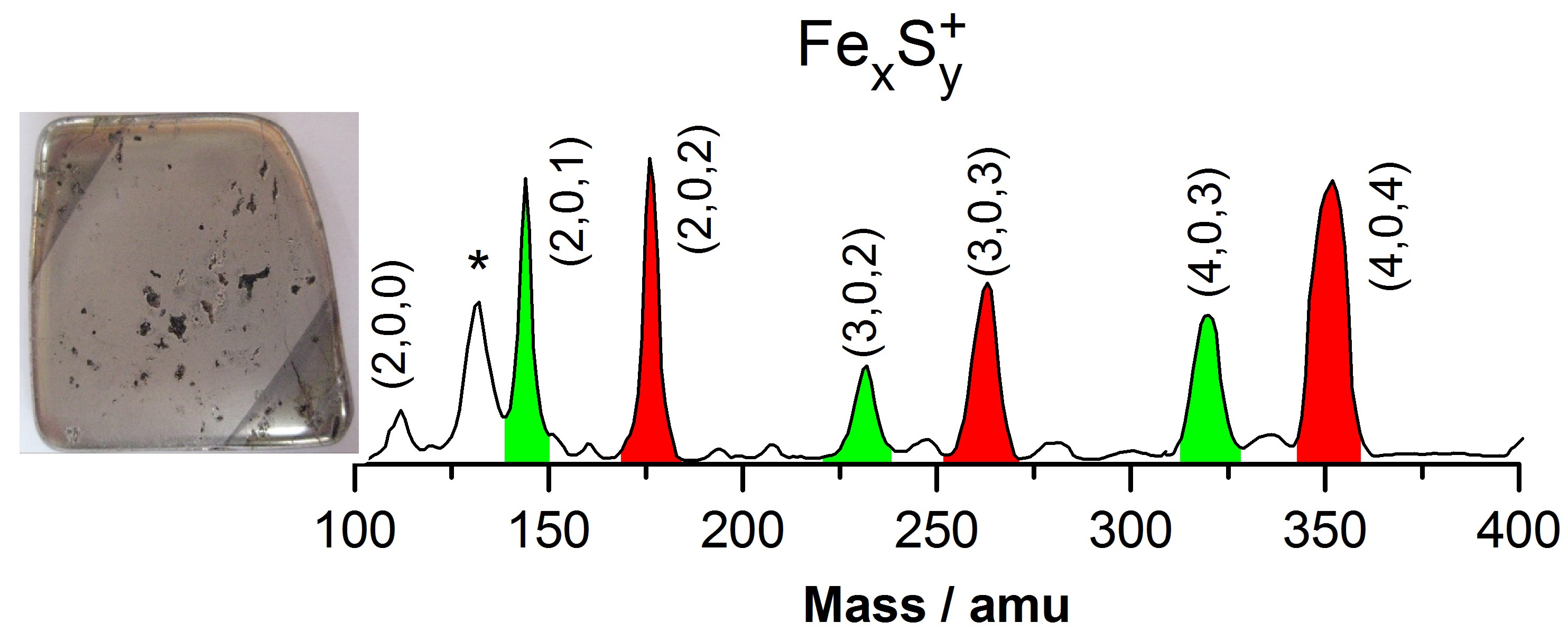
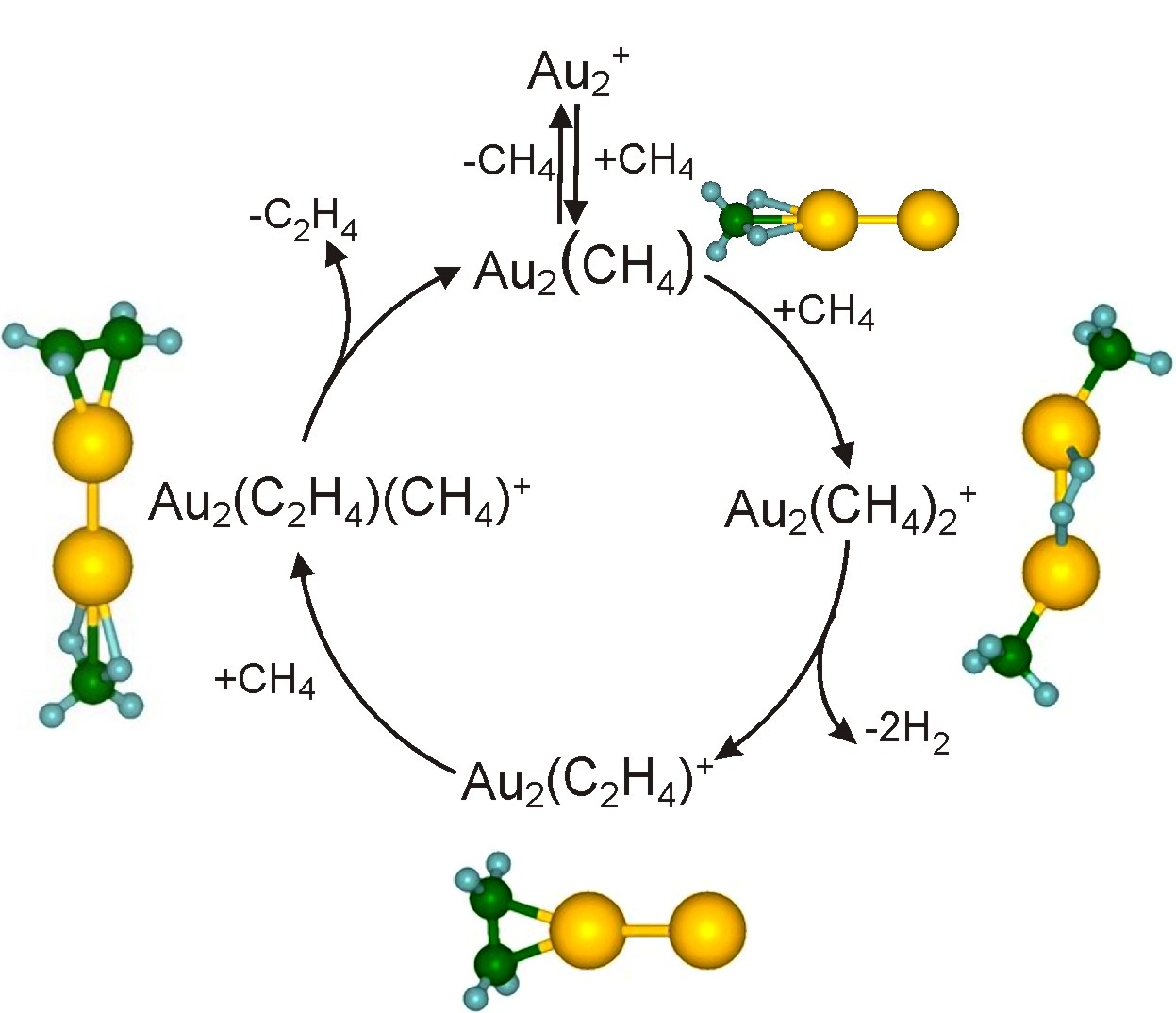
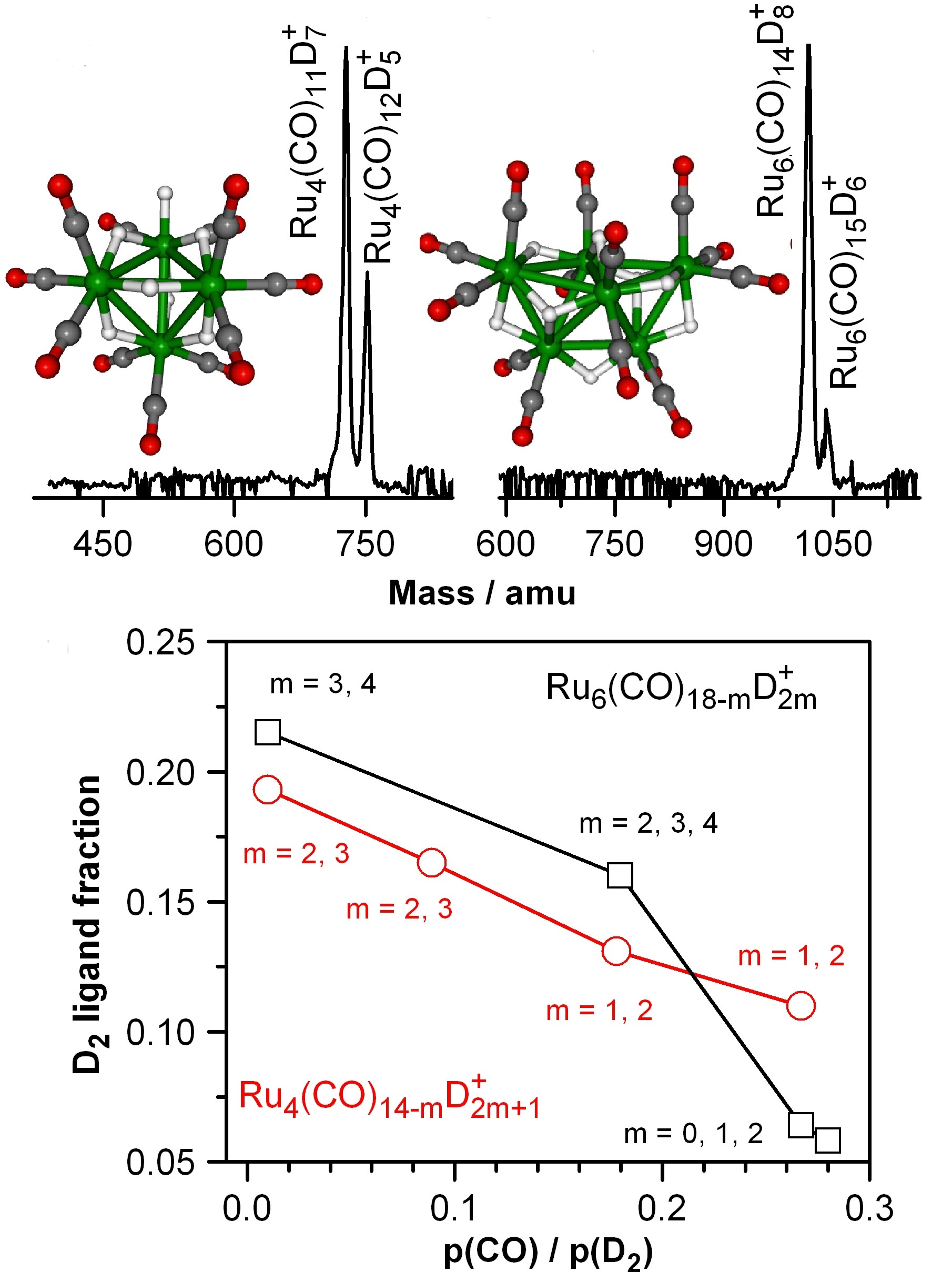
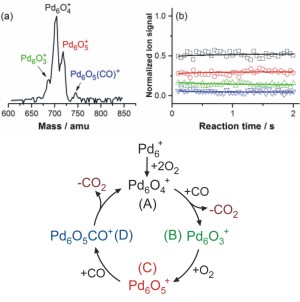
Experimental Methods
Ion trap reactivity experiments
For the detailed investigation of the elementary reaction processes we perform temperature dependent reaction kinetic studies in the gas phase. Clusters of different size are generated by sputtering metal, metal-oxide, or metal-sulfur targets with high energetic xenon ions which are generated in a CORDIS (cold reflex discharge ion source). The produced clusters are thermalized in a quadrupole ion guide Q0 before mass selection in a quadrupole mass filter Q1. The cluster ion beam containing only clusters of one specific mass is then transferred via Q2 into the temperature variable (20-300K) octopole ion trap, which is prefilled with helium buffer gas and a small, well defined fraction of reactants. After a chosen reaction time, all ionic reactants, intermediates, and products are extracted from the ion trap, and the ion distribution is analyzed via a second quadrupole mass filter Q3. By recording all ion intensities as a function of the reaction time kinetic data can be obtained which allows for the determination of reaction mechanisms and the corresponding rate constants.
More details can be found in:
Infrared Multi-Photon Dissociation (IR-MPD) Spectroscopy
For a more detailed insight into the metal cluster mediated activation of small molecules we additionally perform infrared vibrational spectroscopic experiments. For these experiments we use the light provided by the free electron laser FELIX or the free electron laser for intracavity experiments FELICE, both loacted at the FELIX laboratory in Nijmegen, The Netherlands. This method allows for detailed information on the geometric structure of the formed reaction intermediates and final products as well as information on bond activation processes.